What's New Archive
New Puget Sound Marine Waters 2020 Report Released: Automated buoys and volunteers helped gather critical Puget Sound data during pandemic. Photo Credit: Puget Sound Partnership 2021
New Report Released: Automated buoys and volunteers helped gather critical Puget Sound data during pandemic
On October 27, the Puget Sound Marine Waters Work Group of the Puget Sound Ecosystem Monitoring Program released the tenth annual report on marine water conditions in Puget Sound providing a comprehensive long-term view and current assessment of the Puget Sound marine ecosystem. There were few extreme weather or ecological events in 2020, but overall, conditions in Puget Sound were generally warmer, sunnier, and wetter than in typical years. The report further reveals patterns and trends in numerous environmental parameters, including plankton, water quality, climate, and marine life. The observations in this report collectively provide both a comprehensive long-term view and current assessment of the Puget Sound marine ecosystem.
In Puget Sound, ocean acidification (OA) continues as does our understanding of patterns. Annual average atmospheric carbon dioxide (CO2) values over Hood Canal were high relative to globally averaged marine surface air, yet were at the same level as in 2019. OA in Puget Sound is of particular concern as estuarine processes, both natural and human-mediated, can also increase the CO2 content and lower the pH of marine waters. Moreover, coastal upwelling brings deeper waters with naturally higher CO2 concentrations upwards and into Puget Sound via the Strait of Juan de Fuca. Thus, Puget Sound is influenced by a variety of drivers that exacerbate the growing OA signal, making our waters particularly sensitive to these conditions. All of these changes have ramifications for marine food webs and are areas of active current research, including PMEL's Moored Autonomous pCO2 (MAPCO2TM) system collecting on atmospheric and surface seawater xCO2 (mole fraction of CO2) at the Ćháʔba· mooring off of La Push, WA and at the Cape Elizabeth mooring.
Having high-quality observations of carbon in the coastal environment is important for understanding coastal ocean carbon and its impact throughout the water column and the ecosystem. Learn more about PMEL's Carbon and Ocean Acidification Research.
Read the full report here.
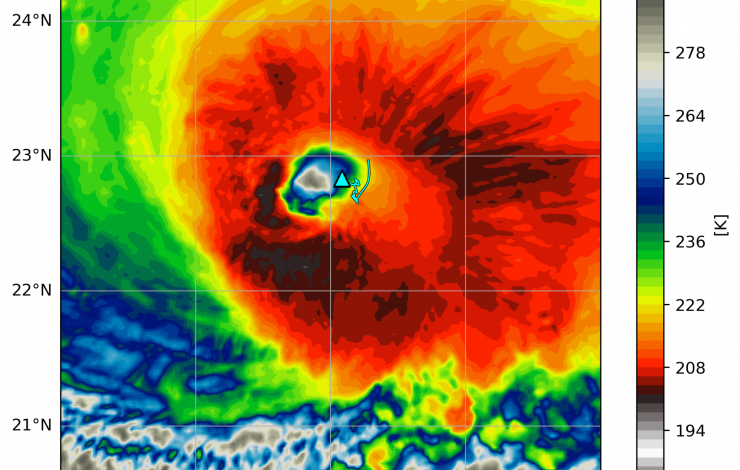
Saildrone 1045 location (triangle) overlaid on an infrared image from NOAA geostationary satellite (colors) at 16:00 UTC September 30, 2021. The saildrone track (light blue line) starts at 04:00 UTC on the same day. Courtesy of Edorado Mazza/University of Washington.
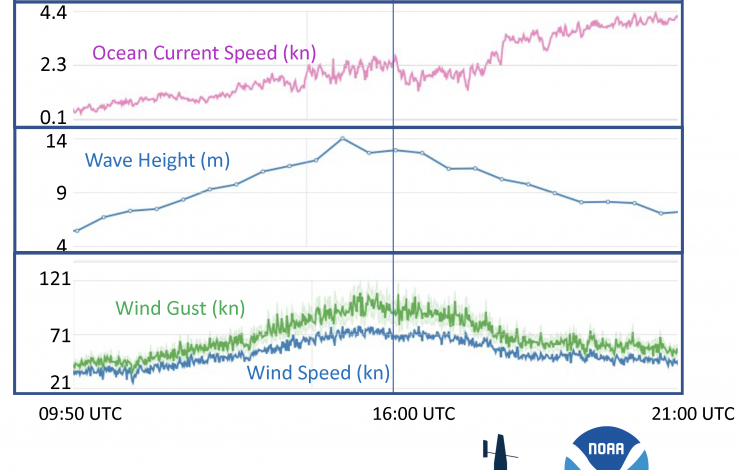
Time series of saildrone measurements of wind, humidity, pressure, current speed, wave heights and solar radiation on September 30, 2021. Vertical line marks the time when the saildrone was located inside the eyewall of Hurricane Sam. Click on image to view all the variables.
Saildrone 1045, one of the five drones PMEL and AOML deployed in the western Atlantic was steered into position to intercept Category 4 Hurricane Sam on September 30, 2021 to record critical data. The encounter required precise interpretation of hurricane forecasts and tight collaboration with Saildrone pilots. The saildrone measured one-minute sustained wind speeds of up to 90.6 miles per hour (40.5 m/s) with wind gusts up to 126.5 mph (56.5 meters/second), waves exceeding 46.4 feet (14.2 meters), and saturated air near the surface. The saildrone was in the hurricane eye next to the eyewall at 16:00 UTC / 12:00 EDT and was in sustained hurricane-force winds (at least 75 mph) for about two hours straight. The first ever drone camera images and video show a large amount of sea spray from the ocean surface and are a frightening display of the power of tropical cyclones.
This is the first time that sea surface conditions near a hurricane eye were observed by an uncrewed surface vehicle. Its success is built upon years of partnership between NOAA and Saildrone, Inc. with testing in progressively more hostile environments and 5 years of innovative engineering on the platform, sensors, and data processing, months of preparation leading to the deployment, and dedicated efforts of operating saildrones by the NOAA science team and Saildrone pilots during the deployment. This animation shows how the saildrone intercepted Hurricane Sam, and this animation, created by scientists at NOAA AOML, shows how the saildrone was positioned to intercept Hurricane Sam alongside saildrone observations of Hurricane Sam.
Prior to hurricane Sam, the NOAA saildrones also measured tropical storms Peter (9/21/2021), Henri (8/20/2021), Grace (8/17/2021) and Fred (8/13/2021). Read more about these encounters on the mission blog: https://www.pmel.noaa.gov/saildrone-hurricane2021/mission_blog.html
Read more on NOAA.gov: A world first: Ocean drone captures video from inside a hurricane
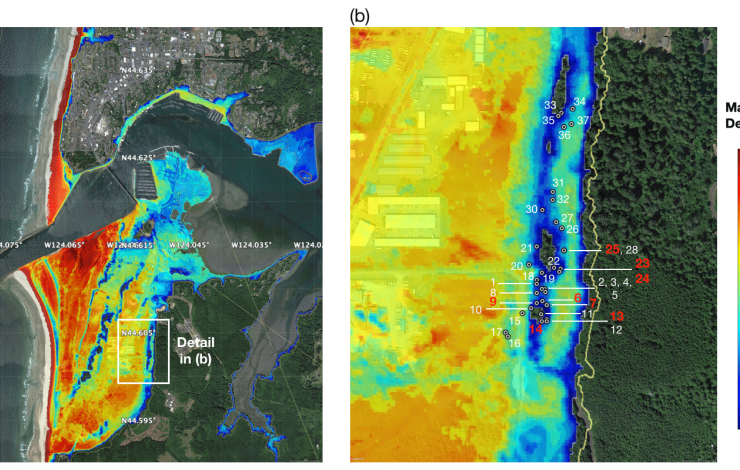
The left panel shows the model of maximum tsunami inundation depth at South Beach for the 1700 CE event and on the right a zoomed-in view of the tsunami inundation depth at Mike Miller State Park. Gray dotted circles show location of trees used in this study on the north side of the stand. Colors on the map show inundation depth from the model, implying 0–10 m of inundation depth at the Mike Miller Park Douglas-fir stand. Green areas are high ground locations that show no inundation. Dziak et al. 2021
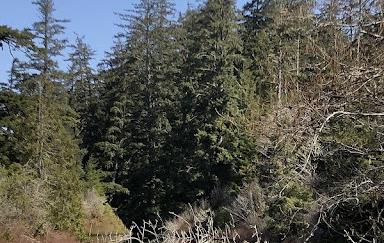
A stand of old growth Douglas-firs near a pond in Mike Miller Park in South Beach, Oregon
Core samples taken from a stand of old growth Douglas-fir trees in the South Beach area just south of Newport showed reduced growth following the 9.0 earthquake and subsequent tsunami that struck the Pacific Northwest in 1700.
The physical evidence from the Douglas-fir tree rings confirms modeling that depicts the reach of the January 1700 quake, which was the last major earthquake to hit the Cascadia Subduction Zone, said Robert Dziak, NOAA PMEL Acoustic Program lead.
“The tsunami appears to be the event that most affected the trees’ growth that year,” said Dziak, whose work includes ocean acoustic studies, signal analysis and tsunami modeling. He also holds a courtesy appointment in Oregon State University’s College of Earth, Ocean, and Atmospheric Sciences. “Getting these little bits of the picture helps us understand what we might expect when the next ‘big one’ hits.”
The findings were published recently in the journal Natural Hazards and Earth System Sciences.
The idea for the study dates back more than a decade; Dziak was aware of past research that had shown evidence of the 1700 quake in trees in Washington, and thought it might be worth seeing if similar evidence existed in Oregon.
The first challenge was finding a stand of old growth Douglas-firs in the tsunami inundation zone. The researchers looked at a few places before locating the stand in Mike Miller Park in South Beach, about two kilometers south of Yaquina Bay and 1.2 kilometers east of the present-day ocean shoreline.
“We’re not sure why this tree stand wasn’t logged over the years, but we’re very fortunate to have a site so close to the coastline that has survived,” said coauthor Bryan Black of the Laboratory of Tree-Ring Research at the University of Arizona, Tucson.
A new and updated tsunami model run by the researchers as part of the study shows that the area could have been inundated by up to 10 meters of water in the 1700 tsunami event, said Dziak.
Once the old growth stand was identified, the researchers collected core samples from about 38 trees using a process that allows them to analyze the tree rings without damaging the overall health of the trees. The majority of the trees dated to around 1670, with one dating to 1650, Dziak said.
They analyzed the growth rates in the rings and compared the growth rates to those of other old-growth Douglas-firs at sites not in the tsunami inundation zone. They found that in 1700 the trees in the tsunami inundation zone showed a significantly reduced growth rate.
Researchers are still working to figure out why the tsunami might have affected the trees’ growth since the trees are relatively far from the shoreline. They suspect it may be a combination of the ground shaking from the earthquake and the inundation of seawater.
“The salty seawater from a tsunami typically drains pretty quickly, but there is a pond area in Mike Miller Park where the seawater likely settled and remained for a longer period of time,” Dziak said.
Black added that the researchers’ next step is to conduct an isotopic analysis on the wood from 1700.
“We will look for signatures consistent with those found in trees that were inundated by the 2011 Tohoku tsunami in Japan,” he said. “If successful, we could develop a powerful new technique to map prehistoric tsunami run-up along the Pacific Northwest coast.”
Yong Wei of the University of Washington Cooperative Institute for Climate, Ocean and Ecosystem Studies and Susan Merle of the Cooperative Institute for Marine Resource Studies at Oregon State University’s Hatfield Marine Science Center are co-authors.
Originally posted on OSU News on August 24, 2021
After spending 8 months under water and ice, two of NOAA’s three Arctic-deployed pop-up floats have successfully surfaced in the Chukchi Sea and are transmitting data on temperature, pressure, photosynthetic active radiation (PAR), and chlorophyll fluorescence. These data are from measurements taken while the float is anchored to the seafloor, while it rises through the water column, and while it is trapped under the ice at the water-ice boundary.
The two floats were initially deployed in the fall of 2020 by the scientists and crew of NOAA Ship Oscar Dyson. These are part of the 4th cohort of deployments since 2017, and the second cohort deployed in the Chukchi Sea. The first float to surface has transmitted data from the four months it spent on the seafloor, just over a month of data from when it was trapped under ice, and about half of the photos it took while under ice. The second float has transmitted about five months of data from the seafloor, just over four months of data from under the ice along with the under-ice photos it captured, and 7 days of open ocean sea surface temperature.
Pop-up floats provide an inexpensive method to explore a unique micro-ecosystem under floating ice. The floats collect ocean health data to help researchers better understand the rapidly changing Arctic ecosystem. They collect data during the ice-covered winter and spring months, a time during which it isn’t possible for researchers to penetrate the ice from above to study the water underneath. The water column data they collect on their rise up from seafloor to surface is essential for researchers to monitor ongoing ecosystem changes in the Arctic, such as watching for harmful algal blooms and documenting biodiversity in the environment. The floats can also help researchers measure the extent of the cold pool, an area of cold water about 30 meters deep that results from melting Arctic ice from the previous season.
NOAA PMEL began development of these floats in 2015. The float is an orange sphere equipped with sensors to measure temperature, pressure, and other ocean conditions as well as cameras to capture under ice imagery. It is deployed during the ice-free summer months and anchors to the seafloor measuring bottom-ocean conditions. It stays here collecting measurements throughout the winter and early spring. It then rises up through the water column at a pre-programmed time, in this case early March to mid-April, capturing data at various depths to create a profile of the water column. The floats are then trapped under ice when it reaches the surface, and will continue collecting data and images of conditions at the water-ice boundary. When the ice melts in mid-May and early June, the floats can emerge fully from the ice to reach the surface and begin transmitting their stored data, while continuing to monitor sea surface temperature.
Read more about the 2020 pop-up deployments in Arctic Today and on the ITAE website.
On August 9, 2021, representatives from NOAA and the Ministry of Earth Sciences (MoES) of India signed an updated partnership agreement that marks more than 12 years of partnership between these nations in the name of ocean and atmospheric observations for improved weather and climate prediction. The virtual signing ceremony included a live demonstration of a new joint oceanographic data portal that makes data from the RAMA-OMNI moored buoy array in the Indian Ocean publicly available for the benefit of global science, forecasting, and disaster preparedness.
“The Indian Ocean is where the monsoon patterns arise that will impact the agriculture and the economic activity of India. But it’s the same basin as well where weather begins that affects North America,” said Craig McLean, NOAA Assistant Administrator for Oceanic and Atmospheric Research. “Our weather in the Americas, the farmlands and what we declare as the production belt of wheat and other crops in the United States - our Midwest - it’s told in advance by understanding what is happening in the Indian Ocean.”
The Research Moored Array for African-Asian-Australian Monsoon Analysis and Prediction (RAMA) was designed to study the Indian Ocean’s role in monsoons, improve monsoon forecasts, and to better study the historically data-sparse Indian Ocean. The first RAMA buoys were deployed by NOAA PMEL in 2004, with the most recent moorings deployed in the Arabian Sea in 2019. MoES has extended operational support to the RAMA array since the earliest deployments. RAMA buoys collect data on a range of atmospheric and oceanic variables, including ocean temperature, salinity, currents, wind, sea level air pressure and humidity. Data collected within RAMA has been extensively used globally and in collaborations between NOAA and MoES scientists to understand the thermohaline structure in the Bay of Bengal, and to validate satellite products and ocean-climate-weather models.
The Ocean Moored Buoys in the Northern Indian Ocean (OMNI) array was initially deployed in 2012, and MoES announced in 2018 that data from the open ocean elements of the OMNI array would be open access and shared freely among researchers to help improve the accuracy of forecasts.
The renewed partnership and joint data portal will significantly benefit the U.S., Indian, and global community working in ocean and climate science, forecasting, and disaster preparedness, especially as we enter the UN Decade of Ocean Science for Sustainable Development and look for new ways to address the climate crisis.
Story modified from NOAA’s Global Ocean Monitoring and Observing Program.

The research study calculated the percent change from the ’80s and ’90s to the 2010s in the nighttime vapor pressure deficit, a measure of the drying power of the air, during summer months. Nighttime conditions were much more conducive to drying in recent years, especially in California’s central valley and the Bitterroot-Blue Mountain region of Idaho and surrounding states. Chiodi et al. 2021/Geophysical Research Letters
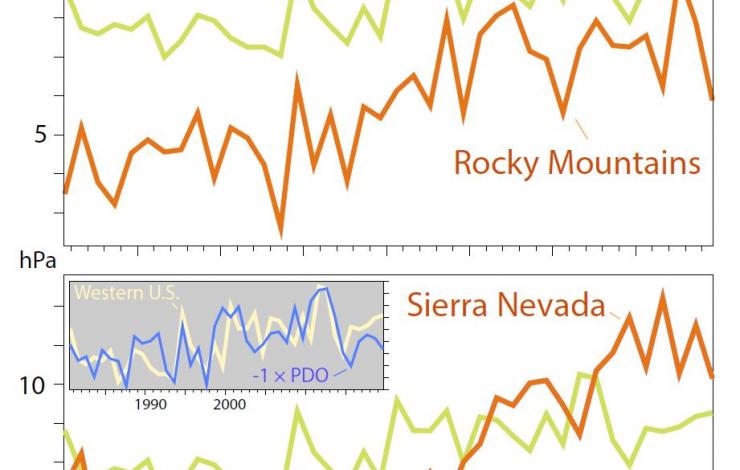
Focusing on the two areas with the biggest change in nighttime air — the Northern Rockies (orange line at top) and the southern Sierra Nevada (orange line at bottom) — shows much bigger changes in summer nighttime vapor pressure deficit than the average across the West (green line). The study finds a link between the average value across the West and the Pacific Decadal Oscillation, a long-term climate pattern shown in the inset.
Firefighters have reported that Western wildfires are starting earlier in the morning and dying down later at night, hampering their ability to recover and regroup before the next day’s flareup.
A study by NOAA Cooperative Institute at the University of Washington and U.S. Forest Service scientists shows why: The drying power of nighttime air over much of the Western U.S. has increased dramatically in the past 40 years. The paper was published online in July in Geophysical Research Letters.
“Nighttime is an important time in fire management. When fires die down at night it gives firefighters a chance to rest, move equipment and strategize. The problem firefighters are reporting is an unexpected increase in nighttime fire activity,” said lead author Andy Chiodi, a UW research scientist at the Cooperative Institute for Climate, Ocean & Ecosystem Studies and at NOAA's Pacific Marine Environmental Laboratory . “Our findings support that this has been going on over the last 40 years over much, but not all, of the Western U.S.”
Earth’s atmosphere is warming due to climate change, and warming in many places has been greater at night. Warmer night air had been suspected as the culprit altering the daily pattern of wildfire activity, with burns continuing later into the night. The new study, however, shows it’s not just that the night air is warmer, but also a dramatic shift from 1980 to 2019 in its drying power — how much moisture the nighttime air can carry away from the fuels — over much of the Western U.S. This shift is not captured in climate models, and the authors say it could be related to natural long-term cycles rather than to climate change.
“We paid special attention to the change in recent years compared to the conditions seen in the ’80s and ’90s, which is when many of the current firefighters started their careers, and presumably formed their ideas about what normal fire behavior should look like,” Chiodi said. “We tried to quantify the changes that we were hearing about from firefighters.”
Vapor Pressure Deficit
The study looks at the “vapor pressure deficit,” or the difference between the moisture in the air and the saturation moisture level at that air temperature. This difference is a measure of the air’s drying power.
“In the southern Sierra Nevada, the average summer nighttime vapor pressure deficit for the recent decade was 50% higher than the average in the ’80s and ’90s,” Chiodi said. “I was surprised — it’s unusual to see geophysical data change that dramatically.”
Some of this shift in vapor pressure deficit is happening because warmer nighttime air, caused by climate change, produce higher saturation values. But part of the drying power is happening because the nighttime air in some regions has less moisture, and that effect is not predicted by climate change models, at least this much or in this pattern. The authors find a possible connection to the Pacific Decadal Oscillation, a long-term cycle that can influence inland weather.
The increased drying power of nighttime air is especially pronounced in California’s San Fernando Valley and in the Bitterroot-Blue Mountain Region — including parts of the Idaho Panhandle, southeast Washington, northeast Oregon and western Montana.
“Firefighters had been saying for several years that they feel some fires burn later into the evening than they used to,” said co-author Brian Potter at the U.S. Forest Service’s Pacific Wildland Fire Sciences Laboratory. “We found that in some areas, the amount of water in the air is decreasing, sort of doubling up on the warmer nights. These areas, including where the Snake River Complex and Lick Creek fires are burning right now, are much more likely to have fires burn late into the night.”
The analysis used hourly weather outputs from the European Centre for Medium-Range Weather Forecasts. The recently released hourly reconstructions of historical weather allowed investigation of daily cycles. The next step, Chiodi said, is to further explore the causes of these changes in nighttime vapor pressure deficit. After that, he hopes to connect the atmospheric conditions more directly to fuel moisture and fire behavior.
The other co-author is Narasimhan ‘Sim’ Larkin at the U.S. Forest Service’s Pacific Wildland Fire Sciences Laboratory in Seattle. The research was funded by the U.S. Forest Service through its AirFire research team and by NOAA. Story originally posted by the University of Washington.
As the western U.S. experiences record shattering heat waves, mega droughts and the eastern tropical Pacific started its 2021 hurricane season with the earliest tropical storm (Andres) on record going back to the early 1970s, two Uncrewed Surface Vehicle (USV) saildrones were launched on July 23, 2021 from Alameda, CA on a research mission to the eastern tropical Pacific.
The eastern tropical Pacific is a key region for hurricane genesis and El Niño Southern Oscillation (ENSO) development. The ENSO cycle not only modulates hurricane genesis in the eastern tropical Pacific and the tropical Atlantic, but also affects the global marine ecosystem and weather patterns on land. The hurricanes and tropical cyclones generated in the eastern tropical Pacific, whether or not they make landfall, control the critical source of moisture for rainfall, especially over western North America.
This region, however, is a gap in the Tropical Pacific Observing System (TPOS). The two saildrones enroute to the eastern tropical Pacific, will test how USVs may be used to address gaps in the present TPOS. The 150-day mission will target several phenomena including:
- Air-sea interactions and convective development in the eastern Pacific hurricane genesis region
- Air-sea interactions, including carbon dioxide outgassing, in the equatorial upwelling zone
- Wind convergence in the southeastern Inter-Tropical Convergence Zone (ITCZ) between 2°S and 5°S, often referred to as “the double ITCZ” region due to common biases of this phenomenon in models
- Air-sea interactions in the frontal zone north of the cold equatorial upwelling; and
- Contrasting subtropical and tropical oceanic and atmospheric states in the eastern Pacific.
The mission is funded in part by NOAA Office of Marine and Aviation Operations (OMAO), NOAA Global Ocean Monitoring and Observing Program (GOMO), and NOAA National Oceanographic Partnership Program (NOPP) bringing together partners across NOAA, universities, and industry, along with international partners from Mexico and France. Read more about the mission on PMEL's Ocean Climate Stations page.
On June 13, scientists aboard the NOAA Ship Ronald H. Brown set out on the West Coast Ocean Acidification Research Cruise to characterize conditions along the West Coast of North America and continue to build a unique time-series of carbon and hydrographic measurements in areas expected to be highly impacted by ocean acidification. Scientists have been collecting samples from CTDs, collecting plankton and water samples for genomics analysis, and conducting the first systematic regional survey of methane gas coming out of the thousands of seeps along the west coast.
The California Current System, running along the North American west coast from British Columbia to Baja California, is a region where seasonal upwelling brings nutrient- and carbon dioxide-rich and oxygen-poor waters to the surface. Increasing levels of carbon dioxide from upwelling and anthropogenic emissions, cause a series of chemical reactions that are ultimately increasing acidity in these waters. Because it is an area with high rates of primary production by phytoplankton, air-sea carbon dioxide exchange, and carbon export to the open ocean and sediments, it is particularly susceptible to the impacts of ocean acidification and hypoxia. Understanding the progression of ocean acidification in coastal areas in the context of these other natural processes is critical for developing management, mitigation, and adaptation strategies.
This 47-day research cruise brings together an international team of scientists from the United States, Canada, Mexico, Finland, and the Netherlands to measure acidity, temperature, oxygen, and chlorophyll from 16 transect lines stretching from British Columbia, Canada to San Diego, California. They also have deployed net tows to sample phytoplankton, zooplankton, and fish to analyze how the marine food web is being affected by acidified waters.
With data collected from this cruise, and previous ocean acidification cruises in this region, scientists are documenting the changing ocean acidification conditions and how they are impacting marine ecosystems against a backdrop of multiple stressors including warming and deoxygenation. In 2016, measurements from this cruise demonstrated, for the first time, that ocean acidification along the US Pacific Northwest coast is impacting the shells and sensory organs of some larval Dungeness crab and that pteropods sampled near the coasts of Washington and Oregon had shells 37 percent thinner than those in waters further offshore.
With the comprehensive approach taken on this year’s mission- combining detailed physical, chemical and biological measurements - we will not only better understand how our ocean is changing, but also test what new tools can be used to assess the future of these important marine ecosystems.
“A strong understanding of both the changes in chemistry and marine life allows us to make informed decisions to sustain the ecosystems, communities and industry members along the West coast,” says Libby Jewett, Director of NOAA’s Ocean Acidification Program which funds this research cruise.
Follow along scientists aboard with NOAA’s Ocean Acidification Program’s Twitter, Instagram and Facebook .
Atmospheric cold pools over the tropical oceans produce large changes in air temperature and wind speed in the planetary boundary layer. But how they affect the larger atmospheric circulation is not clear. Cold pools are pockets of air cooler than the surrounding environment that form when rain evaporates under thunderstorms. These relatively dense air masses, ranging between 10 to 200 kilometers in diameter, lead to downdrafts that, upon hitting the ocean surface, produce temperature fronts and strong winds that impact the surrounding environment. To understand the role of cold pools in tropical convection, scientists need detailed measurements of these events; however, observations in hard-to-reach ocean locations have been lacking.
Uncrewed surface vehicles, or USVs, could be a solution. In a new study published in Geophysical Research Letters, scientists from the University of Washington and NOAA’s Pacific Marine Environmental Laboratory describe the use of Saildrone USVs, wind-propelled sailing drones with a tall, hard wing and solar-powered scientific instruments. Over three multi-month missions between 2017-2019, ten USVs covered over 137,000 kilometers and made measurements of over 300 cold pool events, defined as temperature drops of at least 1.5 degrees Celsius in 10 minutes. In one case, four USVs separated by several kilometers captured the minute-by-minute evolution of an event and revealed how the cold pool propagated across the region.
The Saildrone USVs measured variations in air temperature, wind speed, humidity, pressure, and sea surface temperature and salinity. Analysis of these variables revealed key features of cold pool events, including how much and how quickly air temperatures dropped, how long it took for wind speeds to reach their peaks, and the dynamics of sea surface temperature changes. The results can be used to evaluate mathematical models of tropical convection and explore more questions, like how gusts at cold pool fronts affect air-sea heat fluxes.
These missions are part of a larger effort to enhance the Tropical Pacific Observing System (TPOS) to improve long-term weather forecasts and better understand local and regional implications of global phenomena such as El Niño Southern Oscillation (ENSO), hurricanes, typhoons and marine heatwaves (e.g. the “Blob”). An international team of scientists are working to rethink and refine the TPOS to build a more effective, modern and robust observing system to meet the observational, experimental, and operational needs of today and the future.
Story modified from Eos Original Story posted on July 6, 2021.
NOAA and NASA researchers have found that Earth’s energy imbalance approximately doubled during the 14-year period from 2005 to 2019. Earth's climate is determined by a delicate balance between how much of the Sun's radiative energy is absorbed in the atmosphere and at the surface and how much thermal infrared radiation Earth emits to space. A positive energy imbalance means the Earth system is gaining energy, causing the planet to heat up. The doubling of the energy imbalance is the topic of a recent study, the results of which were published June 15 in Geophysical Research Letters.
Scientists compared data from two independent measurements. NASA's Clouds and the Earth's Radiant Energy System (CERES) suite of satellite sensors measure how much energy enters and leaves Earth's system. In addition, data from a global array of ocean floats, called Argo, enable an accurate estimate of the rate at which the world’s oceans are heating up. Since approximately 90 percent of the excess energy from an energy imbalance ends up in the ocean, the overall trends of incoming and outgoing radiation should broadly agree with changes in ocean heat content.
"The two very independent ways of looking at changes in Earth's energy imbalance are in really, really good agreement, and they're both showing this very large trend, which gives us a lot of confidence that what we're seeing is a real phenomenon and not just an instrumental artifact, " said Norman Loeb, lead author for the study and principal investigator for CERES at NASA's Langley Research Center in Hampton, Virginia. "The trends we found were quite alarming in a sense."
Increases in emissions of greenhouse gases such as carbon dioxide and methane due to human activity trap heat in the atmosphere, capturing outgoing radiation that would otherwise escape into space. The warming drives other changes, such as snow and ice melt, and increased water vapor and cloud changes that can further enhance the warming. Earth’s energy imbalance is the net effect of all these factors. In order to determine the primary factors driving the imbalance, the investigators used a method that looked at changes in clouds, water vapor, combined contributions from trace gases and the output of light from the Sun, surface albedo (the amount of light reflected by the Earth's surface), tiny atmospheric particles called aerosols, and changes in surface and atmospheric temperature distributions.
The study finds that the doubling of the imbalance is partially the result an increase in greenhouse gases due to human activity, also known as anthropogenic forcing, along with increases in water vapor are trapping more outgoing longwave radiation, further contributing to Earth’s energy imbalance. Additionally, the related decrease in clouds and sea ice lead to more absorption of solar energy.
The researchers also found that a flip of the Pacific Decadal Oscillation (PDO) from a cool phase to a warm phase likely played a major role in the intensification of the energy imbalance. The PDO is a pattern of Pacific climate variability. Its fingerprint includes a massive wedge of water in the eastern Pacific that goes through cool and warm phases. This naturally occurring internal variability in the Earth system can have far-reaching effects on weather and climate. An intensely warm PDO phase that began around 2014 and continued until 2020 caused a widespread reduction in cloud coverage over the ocean and a corresponding increase in the absorption of solar radiation.
"It's likely a mix of anthropogenic forcing and internal variability," said Loeb. "And over this period they're both causing warming, which leads to a fairly large change in Earth's energy imbalance. The magnitude of the increase is unprecedented."
Loeb cautions that the study is only a snapshot relative to long-term climate change, and that it's not possible to predict with any certainty what the coming decades might look like for the balance of Earth's energy budget. The study does conclude, however, that unless the rate of heat uptake subsides, greater changes in climate than are already occurring should be expected.
"The lengthening and highly complementary records from Argo and CERES have allowed us both to pin down Earth’s energy imbalance with increasing accuracy, and to study its variations and trends with increasing insight, as time goes on." said Gregory Johnson, co-author on the study and physical oceanographer at the National Oceanic and Atmospheric Administration's Pacific Marine Environmental Laboratory in Seattle, Washington. "Observing the magnitude and variations of this energy imbalance are vital to understanding Earth’s changing climate."
Read the original articles posted by NASA Langley.